Space & Physics
The various avatars of the Hall effect
In this second article of Ed Publica’s series on the Hall effect, Dr. Saraubh Basu examines the physics of the Hall effect variants discovered over the course of the past century.
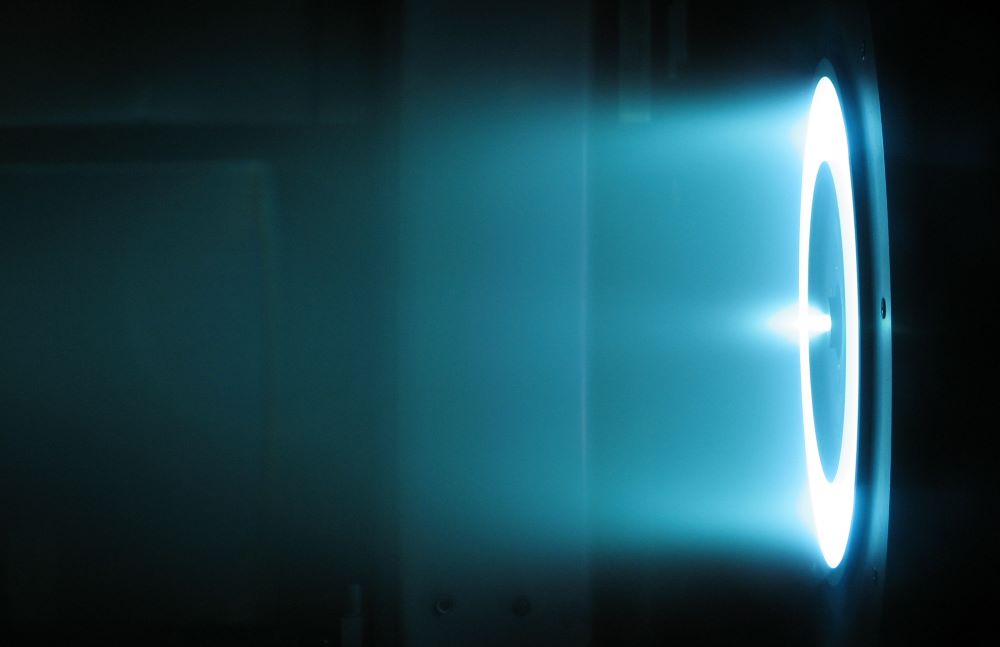
This is the second article of Ed Publica’s series on the Hall effect, which covers the various manifestations of the Hall effect. You can read the first article here.
The ‘anomalous’ Hall effect
In 1881, just two years after Edwin Hall discovered the eponymous Hall effect, he spotted an anomaly when replicating the effect with ferromagnets.
He had observed a tenfold deflection of electric charges this time around, compared to non-magnetic conductors.
Suspecting the magnetic properties played a role, this avatar of the Hall effect is dubbed the anomalous Hall effect. The word ‘anomalous’ is used owing to the fact that external magnetic field no longer remains as a stringent requirement for the Hall effect; instead, the intrinsic magnetization (for instance, the ferromagnet in the above example) fulfils that criterion.
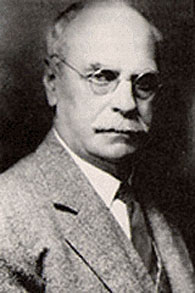
The physicist Edwin Hall. Credit: Wikimedia
The Hall resistivity in ferromagnets increase steeply under the presence of very weak magnetic fields. However, in stronger magnetic fields, the Hall resistivity doesn’t increase further very much. This saturation is rather strange, for it is in contrast to the classical Hall effect where the Hall resistivity maintains its steady growth.
There are several other effects that play a crucial role in determining the anomalous Hall resistivity, thus making it a complicated phenomenon that physicists lack comprehensive understanding about, in comparison to the various other avatars of the Hall effect.
Quantum avatar(s)
The fact that a simple lab experiment showed how the Hall resistivity can be expressed as an equation that contains merely constants, opened up a a plethora of research to understand the cause of this ‘universality’. For it hinted to the involvement of a very fundamental phenomenon.
In 1980, Klaus von Klitzing discovered the quantum avatar of the Hall effect was detected. He was amidst research at a magnetic facility in Grenoble, France, working to improve electron mobility in metal oxide semiconductor field effect transistors (MOSFET). These are transistors that typically operate at extremely low temperatures and under intense magnetic fields.
von Klitzing observed his sample’s Hall resistivity assuming discretized values. This means the resistivity jumps in steps, by a fixed amount that can be scaled as multiples of an integer number (includes 0 along with whole numbers such as 1,2,3, and so on). This discretization reveals the underlying quantum mechanical behavior that has been unraveled at long last – thus bearing its name – the integer quantum Hall effect. von Klitzing later won the Nobel Prize in Physics for 1985 for this work.
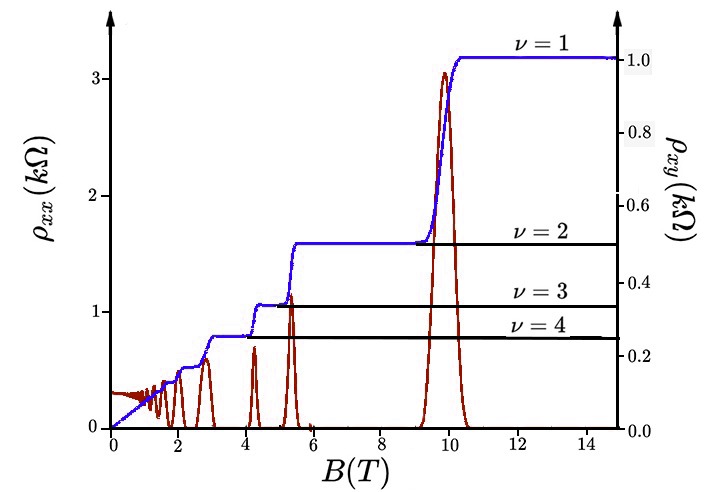
The plot here depicts the transverse and longitudinal Hall resistivity (y-axis) increasing in integer steps as the magnetic field (x-axis) increases. This is due to the integer quantum Hall effect. Credit: Wikimedia
But the quantization isn’t limited to integer multiples. In fact, two years later, the fractional quantum Hall effect was observed in experiments. It was shown there were about 100 fractions, including those that aren’t whole numbers that were now in the formula.
Robert Laughlin, who would later win a share of the 1998 Nobel Prize in Physics, proposed a theory to explain the observations. It boils down to the interaction among electrons, either due to the Coulombic repulsion force or the Pauli exclusion principle.
These interacts would eventually split the degeneracy of these enormously degenerate Landau energy levels. These are quantum states occupied by electrons that complete circular revolutions under the influence of an external magnetic field. Splitting these degeneracies, lead to the opening of an energy gap, for the fractional quantum Hall effect to be observed.
‘Spin’ avatar(s)
Just as there are electric charges in nature, so are there spin currents found in nature. ‘Spin’ is a key property found in quantum particles. Unlike what the name suggests, these quantum particles don’t spin or rotate about any axis passing through them. However, these particles carry an angular momentum as though it does spin.
In 1971, before von Klitzing observed the quantum Hall effect, Mikhail Dyakonov and Vladimir Perel hypothesized the spin Hall effect.
In this avatar of the Hall effect, quantum spins of opposite kinds accumulate at the edges of the sample, orthogonal to the direction in which the charge current passes.
The spin selection can be facilitated by the spin-orbit coupling. This refers to the modified energy levels in an atom when the electron’s motion is under the influence on the magnetic field generated by the nucleus. Strong coupling may be intrinsic to doped semiconductors. The proposal has triggered intense investigation of the phenomenon, with first experimental observations of the spin Hall effect seen in n-doped semiconductors and two-dimensional hole gases.
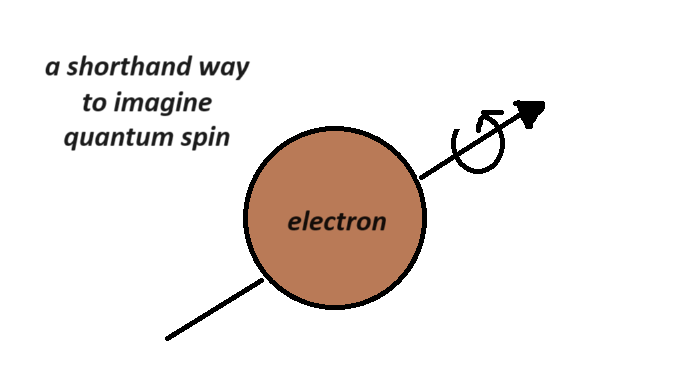
Quantum spins don’t really look like the depiction above, which is meant to showcase a fact that particles like electrons do have an intrinsic angular momentum nonetheless. Credit: Karthik / Ed Publica
For more than a decade, studies concerning the spin current and its application to novel spintronics (or spin electronics) have received plethora of attention. This is with regard to efficiently generating, manipulating and detecting spin accumulation in a sample material. Some progress has also occurred from the device fabrication perspective via techniques such as spin injection, among others.
A major advantage in dealing with the spin current lies in the non-dissipative (or very less dissipation) nature which arises owing to the time reversal invariance of the spin current. This presents a non-dissipative scenario (unlike the dissipative effects seen with charged currents), thus making it quite advantageous for spin transport phenomena.
Furthermore, a quantized version of the spin Hall effect exists, with mercury telluride and cadmium telluride quantum well superlattices, showcasing this effect. In 2005, a quantum treatment was proposed by Charles Kane and Eugene Mele, in the form of a tight binding toy model of electrons operating in a two-dimensional honeycomb lattice.
In fact, the ‘wonder material’ graphene, which is a two-dimensional honeycomb lattice constituting carbon atoms, does satisfy some key requirements for the quantum spin Hall effect. However, it lacks a large spin-orbit coupling among other requirements.
Nonetheless, graphene’s ability to entertain the quantum spin Hall effect, makes it a prospective candidate to find applications in next-generation spintronic devices.
Space & Physics
MIT Physicists Capture First-Ever Images of Freely Interacting Atoms in Space
The new technique allows scientists to visualize real-time quantum behavior by momentarily freezing atoms in motion and illuminating them with precisely tuned lasers
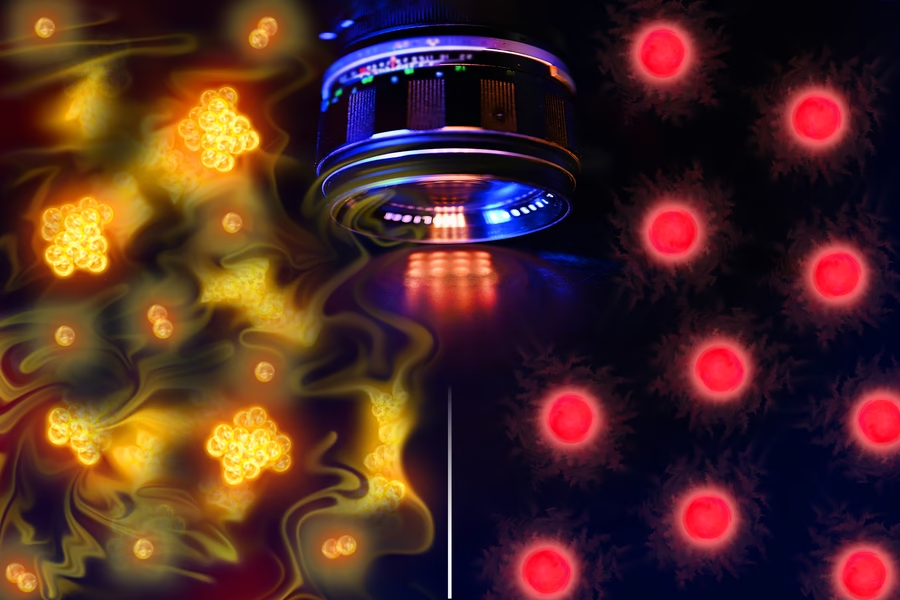
In an intriguing advancement for quantum physics, MIT researchers have captured the first images of individual atoms freely interacting in space — a feat that until now was only predicted theoretically.
The new imaging technique, developed by a team led by Professor Martin Zwierlein, allows scientists to visualize real-time quantum behavior by momentarily freezing atoms in motion and illuminating them with precisely tuned lasers. Their results, published in Physical Review Letters, reveal how bosons bunch together and fermions pair up in free space — phenomena crucial to understanding superconductivity and other quantum states of matter.
“We are able to see single atoms in these interesting clouds of atoms and what they are doing in relation to each other, which is beautiful,” said Zwierlein in a press statement.
Using their method — called “atom-resolved microscopy” — the team was able to trap atom clouds with a loose laser, briefly immobilize them with a lattice of light, and then image their positions via fluorescence. This approach allowed the researchers to observe quantum behaviors at the level of individual atoms for the first time.
The MIT group directly visualized sodium atoms (bosons) bunching together in a shared quantum wave — a vivid confirmation of the de Broglie wave theory — and lithium atoms (fermions) pairing up despite their natural repulsion, a key mechanism underlying superconductivity.
“This kind of pairing is the basis of a mathematical construction people came up with to explain experiments. But when you see pictures like these, it’s showing in a photograph, an object that was discovered in the mathematical world,” said co-author Richard Fletcher in a press statement.
Two other research teams — one led by Nobel laureate Wolfgang Ketterle at MIT, and another by Tarik Yefsah at École Normale Supérieure — also reported similar quantum imaging breakthroughs in the same journal issue, marking a significant moment in the experimental visualization of quantum mechanics.
The MIT team plans to expand the technique to probe more exotic quantum behaviors, including quantum Hall states. “Now we can verify whether these cartoons of quantum Hall states are actually real,” Zwierlein added. “Because they are pretty bizarre states.”
Space & Physics
Indian Researchers Develop Breakthrough Metal-Free Catalyst for Green Hydrogen Production
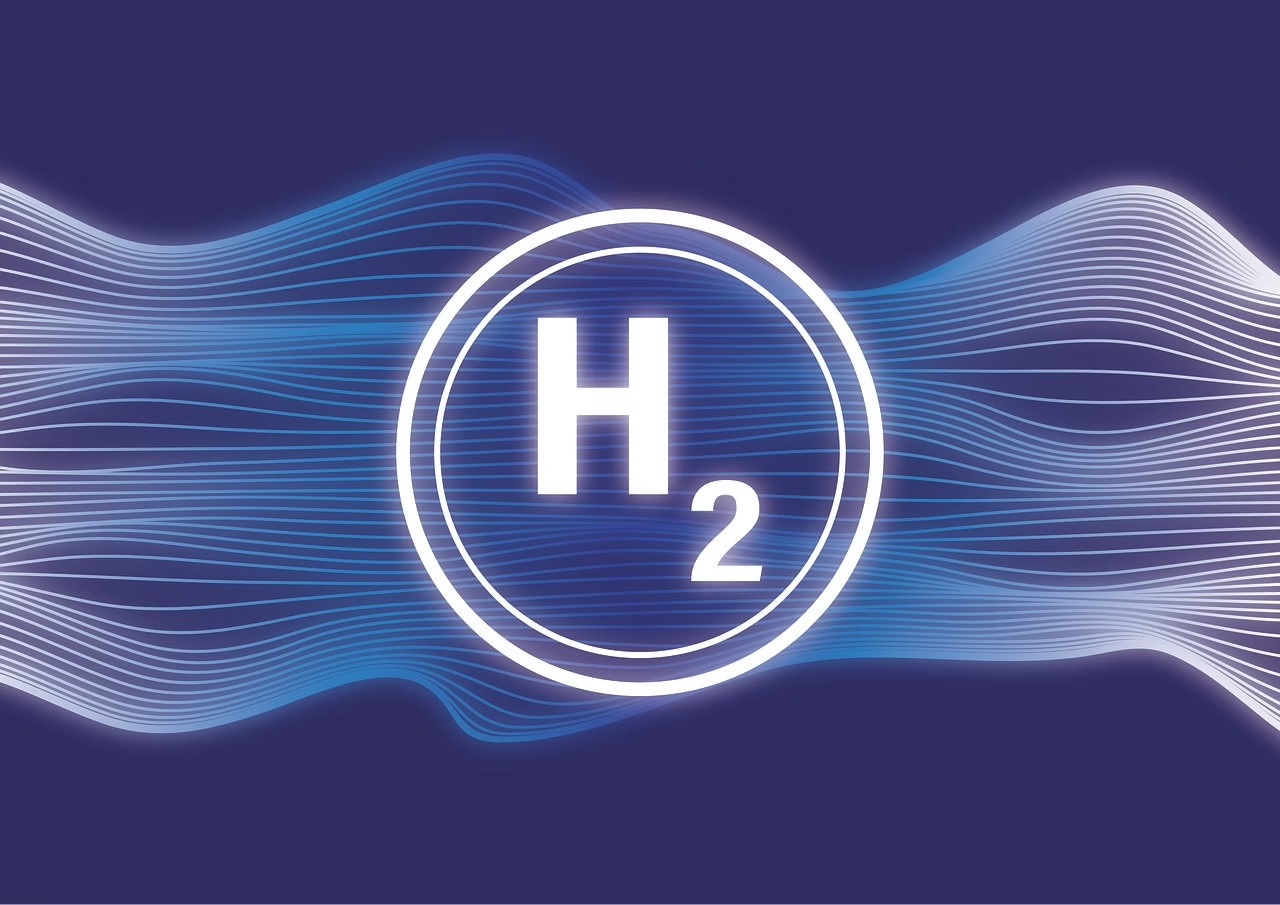
In a major scientific breakthrough, researchers at Jawaharlal Nehru Centre for Advanced Scientific Research (JNCASR), Bengaluru, India, have developed a novel, cost-effective, metal-free porous organic catalyst that enables efficient hydrogen (H₂) production by harnessing mechanical energy. This innovative work could provide a significant boost to India’s National Green Hydrogen Mission and global efforts toward clean energy.
The team, led by Professor Tapas K. Maji from the Chemistry and Physics of Materials Unit at JNCASR—an autonomous institution under the Department of Science & Technology, Government of India—has designed a donor-acceptor-based covalent-organic framework (COF) that functions as a highly efficient piezocatalyst for water splitting. The findings have been published in the journal Advanced Functional Materials.
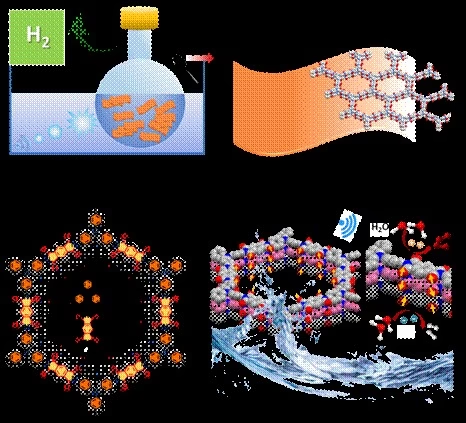
“This discovery breaks the traditional notion of solely employing heavy or transition metal-based ferroelectric materials as piezocatalysts for catalyzing water splitting reaction,” said Professor Maji in a press statement.
The COF, constructed using the donor molecule tris(4-aminophenyl)amine (TAPA) and the acceptor molecule pyromellitic dianhydride (PDA), showcases unique ferrielectric (FiE) ordering. Unlike conventional ferroelectric materials, which have limited surface charge and rapidly reach saturation, this FiE structure dramatically enhances the number of charge carriers within the framework’s porous surface. This enables more effective diffusion and interaction of water molecules, resulting in ultra-high hydrogen production yields.
Prof. Umesh V. Waghmare and his team, also at JNCASR, conducted theoretical analyses confirming that the COF’s unusual electronic structure fosters dipolar ordering, leading to lattice instability and FiE behavior. “These FiE dipoles interact with the flexible twisting molecular motion in the material, making them responsive to mechanical pressure,” said Prof. Waghmare. “As a result, the material can generate electron-hole pairs when mechanically stimulated, making it a highly efficient piezocatalyst.”
The research team also includes Ms. Adrija Ghosh, Ms. Surabhi Menon, Dr. Sandip Biswas, and Dr. Anupam Dey from JNCASR, with significant contributions from Dr. Supriya Sahoo and Prof. Ramamoorthy Boomishankar at IISER Pune, and Prof. Jan K. Zaręba from Wrocław University of Science and Technology, Poland.
The innovation offers a promising alternative to traditional oxide-based piezocatalysts and represents a leap forward in the sustainable production of hydrogen fuel. “The utilization of a cost-effective, metal-free system with a high production rate of H2 by harvesting mechanical energy opens up a new route to green H2 based on porous heterogeneous catalysts,” added Prof. Maji.
Space & Physics
Engineers Edge Closer to Practical, Fault-Tolerant Quantum Machines
The findings demonstrate the foundational physics needed to achieve ultra-fast quantum readout, an essential step toward scalable and fault-tolerant quantum systems
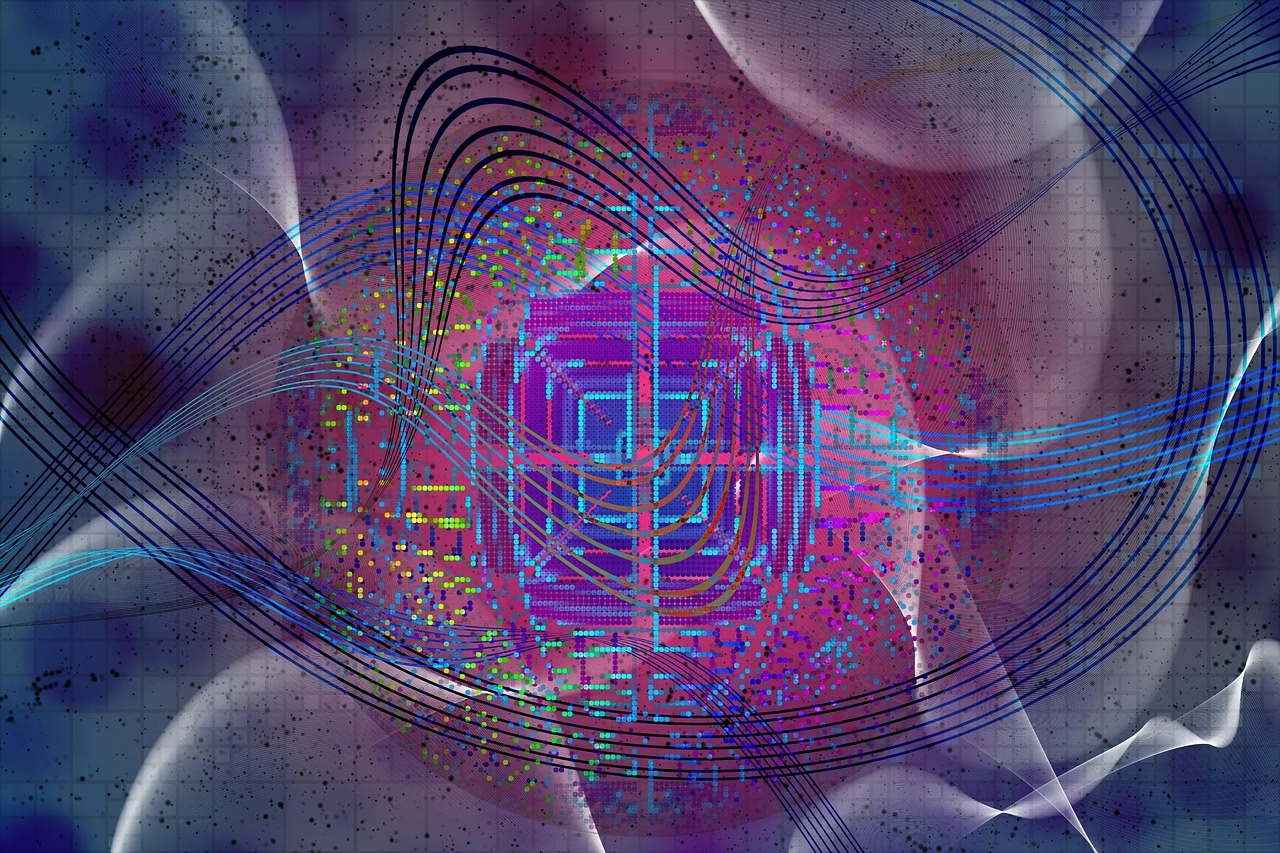
In a breakthrough that could accelerate the future of quantum computing, researchers at MIT have demonstrated the strongest nonlinear light-matter coupling ever recorded in a quantum system — a development that may enable quantum operations and measurements in mere nanoseconds.
This leap forward hinges on a novel superconducting circuit design featuring a device called the quarton coupler, invented by lead researcher Yufeng “Bright” Ye, PhD ’24. The technology enables interaction between photons (particles of light that carry quantum information) and artificial atoms (units that store quantum data), which is central to the speed and accuracy of quantum computers.
“Usually, you have to measure results between rounds of error correction, and slow readout can become a bottleneck,” Ye explained. “This could dramatically accelerate progress toward fault-tolerant quantum computing and practical real-world applications.”
Working with senior author Kevin O’Brien, associate professor and principal investigator at MIT’s Research Laboratory of Electronics, the team connected the quarton coupler to two superconducting qubits on a chip. One served as a photon emitter and the other as a storage atom, enabling extremely strong nonlinear interactions — about ten times stronger than previous demonstrations.
This means a quantum processor could potentially perform tenfold faster operations, allowing scientists to run more quantum error corrections during the brief window when qubits remain coherent. Error correction is essential in quantum computing, where fragile quantum states are easily disrupted.
The team’s findings, published in Nature Communications, demonstrate the foundational physics needed to achieve ultra-fast quantum readout, an essential step toward scalable and fault-tolerant quantum systems.
While this remains a proof of concept, researchers are now working to integrate additional electronic components — such as filters — to build practical readout circuits compatible with full-scale quantum systems. The team also reported success in achieving strong matter-matter coupling between qubits, which could further enhance future quantum operations.
“This isn’t the end — it’s the beginning of a new phase,” said O’Brien. “We now have a powerful physical tool, and the next step is engineering it into something that can be part of a real quantum computer.”
As scientists push toward building large-scale quantum processors, innovations like the quarton coupler bring them closer to unlocking new materials, revolutionizing machine learning, and solving problems beyond the reach of today’s fastest supercomputers.
-
Earth2 months ago
How IIT Kanpur is Paving the Way for a Solar-Powered Future in India’s Energy Transition
-
Space & Physics6 months ago
How Shyam Gollakota is revolutionizing mobile systems and healthcare with technology
-
Space & Physics1 month ago
Could dark energy be a trick played by time?
-
Society2 months ago
Starliner crew challenge rhetoric, says they were never “stranded”
-
EDUNEWS & VIEWS5 months ago
“One Nation, One Subscription” is a welcome step, in light of publishers’ apathy
-
Space & Physics4 months ago
Obituary: R. Chidambaram, Eminent Physicist and Architect of India’s Nuclear Program
-
Society6 months ago
Rebranding Bhutan: A case study in transforming identity
-
Know The Scientist6 months ago
Pierre Curie: The precision of a scientific pioneer