Space & Physics
In search for red aurorae in ancient Japan
Ryuho Kataoka, a Japanese auroral scientist, played a seminal role in searching for evidence of super-geomagnetic storms in the past using historical methods
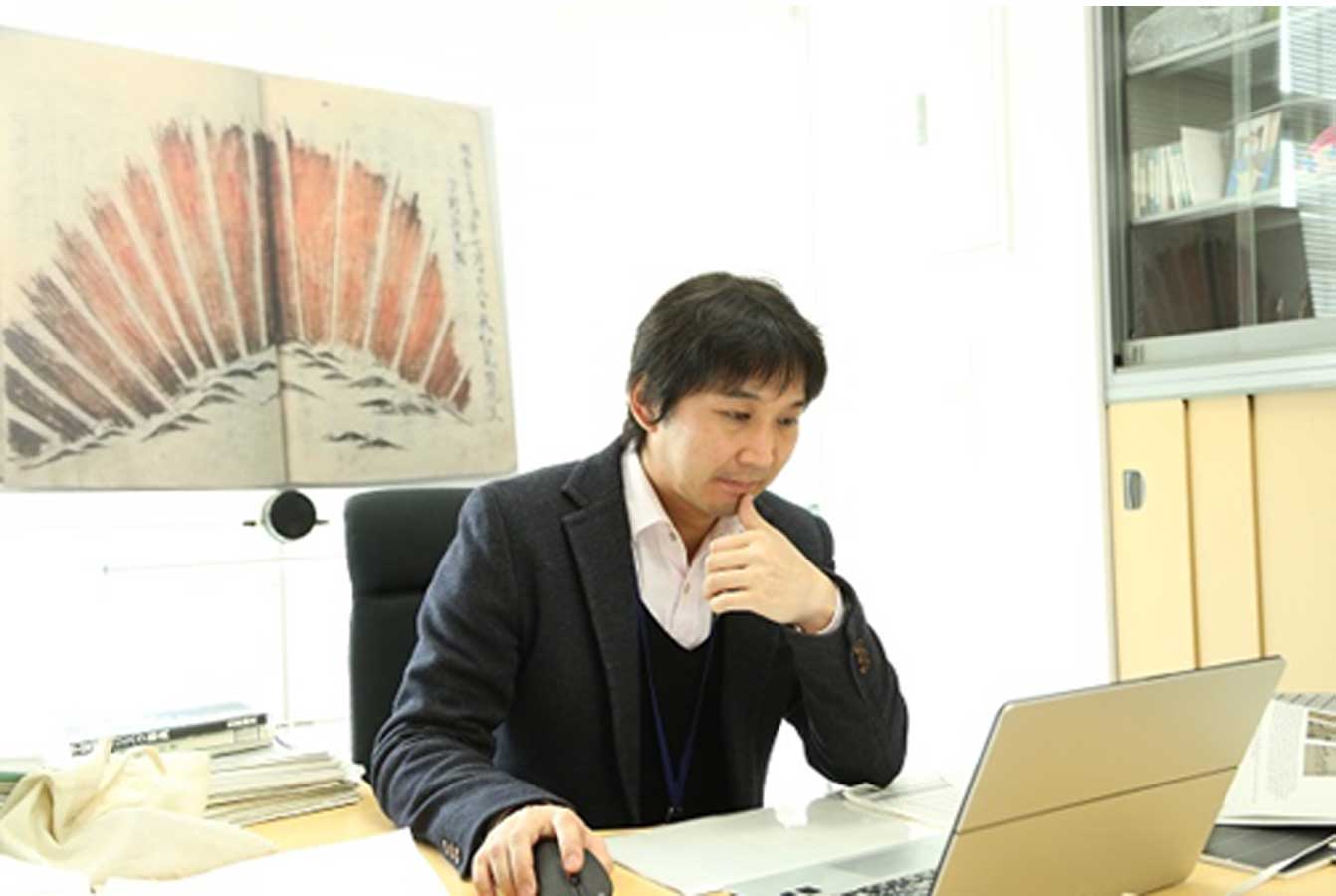
Auroras seen on Earth are the end of a complex process that begins with a violent, dynamic process deep within the sun’s interior.
However, studying the depths of the sun is no easy task, even for scientists. The best they can do is to observe the surface using space-based telescopes. One problem that scientists are attempting to solve is how a super-geomagnetic storm on Earth comes to being. These geomagnetic storms find their roots in sunspots, that are acne-like depressions on the sun’s surface. As the sun approaches the peak of its 11-year solar cycle, these sunspots, numbering in the hundreds, occasionally release all that stored magnetic energy into deep space, in the form of coronal mass ejections (CMEs) (which are hot wisps of gas superheated to thousands of degrees).
Super-geomagnetic storms, a particularly worse form of geomagnetic storm, can induce power surges in our infrastructure, causing power outages that can plunge the world into darkness, and can cause irreversible damages to our infrastructure
If the earth lies in the path of an oncoming CME, the energy release from their resultant magnetic field alignment can cause intense geomagnetic storms and aurorae on Earth.
This phenomenon, which is astrophysical and also electromagnetic in nature, can have serious repercussions for our modern technological society.
Super-geomagnetic storms, a particularly worse form of geomagnetic storm, can induce power surges in our infrastructure, causing power outages that can plunge the world into darkness, and can cause irreversible damages to our infrastructure. The last recorded super-geomagnetic storm event occurred more than 150 years ago. Known as the Carrington event, the storm destroyed telegraph lines across North America and Europe in 1859. The risk for a Carrington-class event to happen again was estimated to be 1 in 500-years, which is quite low, but based on limited data. Ramifications are extremely dangerous if it were to ever happen.
However, in the past decade, it was learnt that such super-geomagnetic storms are much more common than scientists had figured. To top it all, it wasn’t just science, but it was a valuable contribution by art – specifically ancient Japanese and Chinese historical records that shaped our modern understanding of super-geomagnetic storms.
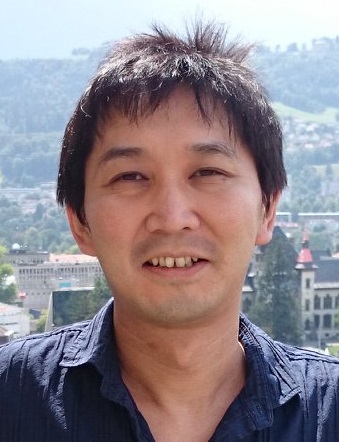
Ryuho Kataoka, a Japanese space physicist, played a seminal role in searching for evidence of super-geomagnetic storms in the past using historical methods. He is presently an associate professor in physics, holding positions at Japan’s National Institute of Polar Research, and The Graduate University for Advanced Studies.
“There is no modern digital dataset to identify extreme space weather events, particularly super-geomagnetic storms,” said Professor Kataoka. “If you have good enough data, we can input them into supercomputers to do physics-based simulation.”
However, sunspot records go until the late 18th century when sunspots were actively being cataloged. In an effort to fill the data gap, Professor Kataoka decided to be at the helm of a very new but promising interdisciplinary field combining the arts with space physics. “The data is limited by at least 50 years,” said Professor Kataoka. “So we decided to search for these red vapor events in Japanese history, and see the occurrence patterns … and if we are lucky enough, we can see detailed features in these lights, pictures or drawings.” Until the summer of 2015, Ryuho Kataoka wasn’t aware of how vast ancient Japanese and Chinese history records really were.
“There is no modern digital dataset to identify extreme space weather events, particularly super-geomagnetic storms,” said Professor Kataoka.
In the past 7 years, he’s researched a very specific red aurora, in documents extending to more than 1400 years. “Usually, auroras are known for their green colors – but during the geomagnetic storm, the situation is very different,” he said. “Red is of course unusual, but we can only see red during a powerful geomagnetic storm, especially in lower latitudes. From a scientific perspective, it’s a very reasonable way to search for red signs in historical documents.”
A vast part of these historical red aurora studies that Professor Kataoka researched came from literature explored in the last decade by the AURORA-4D collaboration. “The project title included “4D”, because we wanted to access records dating back 400 years back during the Edo period,” said Professor Kataoka.
“From the paintings, we can identify the latitude of the aurora, and calculate the magnitude or amplitude of the geomagnetic storm.” Clearly, paintings in the Edo period influenced Professor Kataoka’s line of research, for a copy of the fan-shaped red aurora painting from the manuscript Seikai (which translates to ‘stars’) hangs on the window behind his office desk at the National Institute of Polar Research.
The painting fascinated Professor Kataoka, since it depicted an aurora that originated during a super-geomagnetic storm over Kyoto in 1770. However, the painting did surprise him at first, since he wondered whether the radial patterns in the painting were real, or a mere artistic touch to make it look fierier. “That painting was special because this was the most detailed painting preserved in Japan,” remarked Professor Kataoka. “I took two years to study this, thinking this appearance was silly as an aurorae scientist. But when I calculated the field pattern from Kyoto towards the North, it was actually correct!”
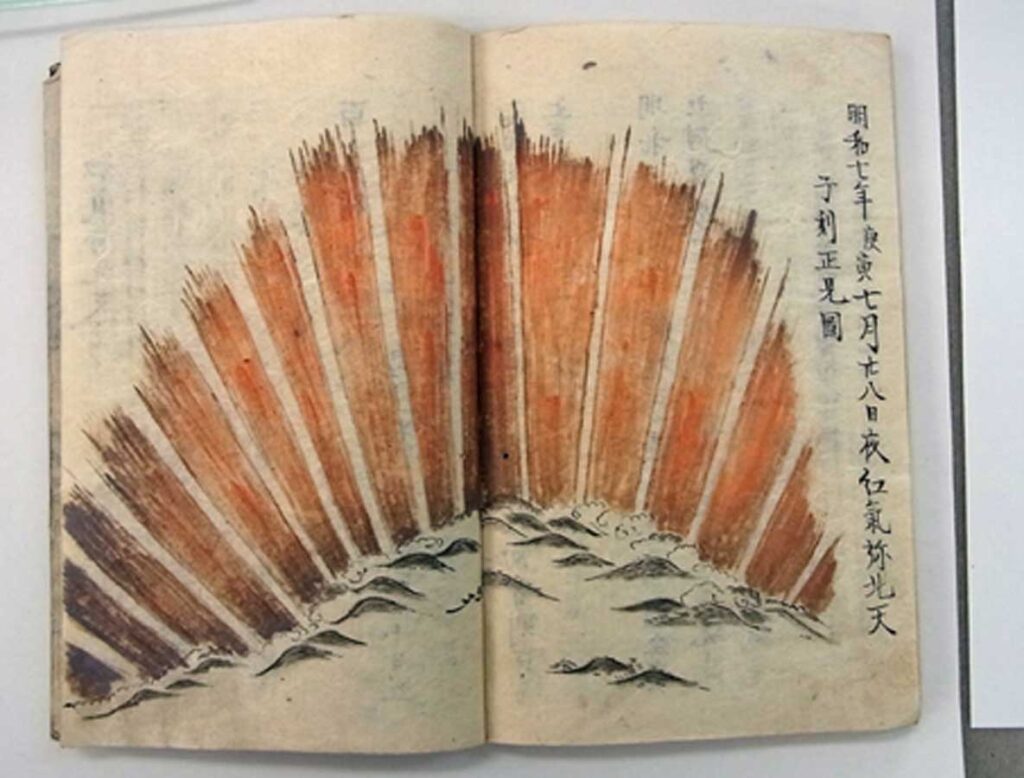
Fan-shaped red aurora painting from the ‘Seikai’, dated 17th September, 1770; Picture Courtesy: Matsusaka City, Mie Prefecture.
The possibility to examine and verify historical accounts using science is also a useful incentive for scholars of Japanese literature and scientists partaking in the research.
“This is important because, if we scientists look at the real National Treasure with our eyes, we really know these sightings recorded were real,” said Professor Kataoka. “The internet is really bad for a survey because it can easily be very fake,” he said laughing. It’s not just the nature in which science was used to examine art – to examine Japanese “national treasures” that is undoubtedly appealing, but historical accounts themselves have contributed to scientific research directly.
“From our studies, we can say that the Carrington class events are more frequent than we previously expected,” said Professor Kataoka. There was a sense of pride in him as he said this. “This Carrington event is not a 1 in 200-year event, but as frequent as 1 in 100 years.” Given how electricity is the lifeblood of the 21st century, these heightened odds do ingrain a rather dystopian society in the future, that is ravaged by a super-geomagnetic storm.
Professor Kataoka’s work has found attention within the space physics community. Jonathon Eastwood, Professor of Physics at Imperial College London said to EdPublica, “The idea to use historical information and art like this is very inventive because these events are so rare and so don’t exist as information in the standard scientific record.”
There’s no physical harm from a geomagnetic storm, but the threat to global power supply and electronics is being increasingly recognized by world governments. The UK, for instance, identified “space weather” as a natural hazard in its 2011 National Risk Register. In the years that followed, the government set up a space weather division in the Met Office, the UK’s foremost weather forecasting authority, to monitor and track occurrences of these coronal mass ejections. However, these forecasts, which often supplement American predictions – namely the National Oceanic and Atmospheric Administration (NOAA) – have failed to specify previously where a magnetic storm could brew on Earth, or predict whether a coronal mass ejection would ever actually strike the Earth.
Professor Kataoka said he wishes space physicists from other countries participate in similar interdisciplinary collaborations to explore their native culture’s historical records for red aurora sightings
The former occurred during the evacuation process for Hurricane Irma in 2017, when amateur radio ham operators experienced the effects of a radio blackout when a magnetic storm affected the communications network across the Caribbean. The latter occurred on another occasion when a rocket launch for SpaceX’s Starlink communication satellites was disrupted by a mild geomagnetic storm, costing SpaceX a loss of over $40 million.
Professor Kataoka said he wishes space physicists from other countries participate in similar interdisciplinary collaborations to explore their native culture’s historical records for red aurora sightings. He said the greatest limitation of the AURORA-4D collaboration was the lack of historical records from other parts of the world. China apparently boasts a history of aurora records longer than Japan, with a history lasting before Christ himself. “Being Japanese, I’m not familiar with British, Finnish or Vietnamese cultures,” said Professor Kataoka. “But every country has literature researchers and scientists who can easily collaborate and perform interdisciplinary research.” And by doing so, it’s not just science which benefits from it, but so is ancient art whose beauty and relevance gains longevity.
Space & Physics
Obituary: R. Chidambaram, Eminent Physicist and Architect of India’s Nuclear Program
Rajagopala Chidambaram (1936–2025), a man whose work shaped the future of modern India, will always be remembered as the chief architect of India’s nuclear journey.
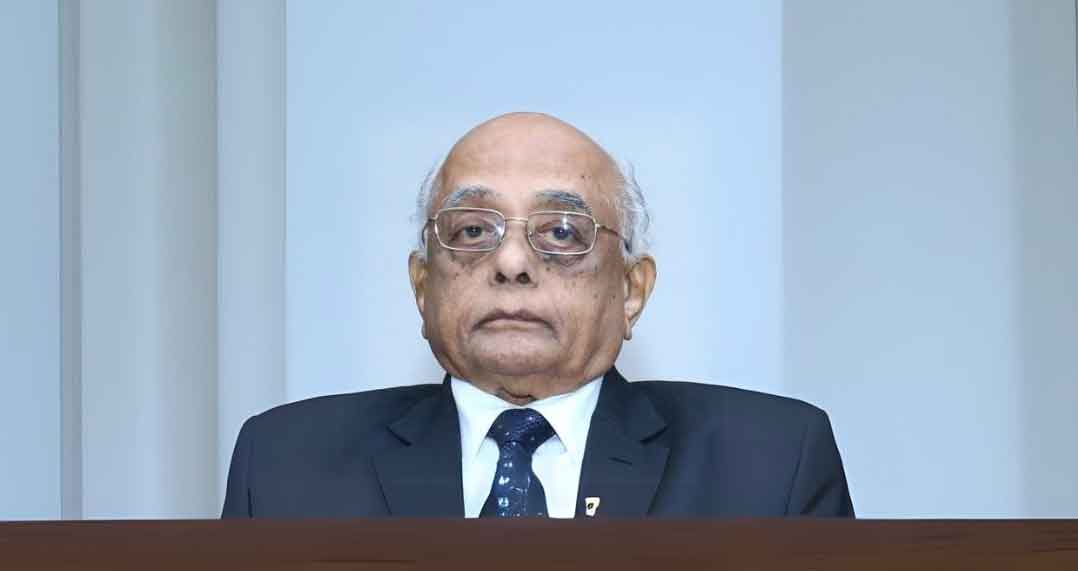
Rajagopala Chidambaram, a world-class physicist and the chief architect of India’s nuclear program, passed away on January 4, 2025, at the age of 88. Renowned for his unparalleled contributions to India’s nuclear defense and energy security, Chidambaram leaves a profound legacy in both the scientific community and the nation’s strategic defense apparatus.
Born on November 11, 1936, in India, Dr. Chidambaram was an alumnus of Presidency College, Chennai, Tamil Nadu, and the Indian Institute of Science, Bengaluru, Karnataka. His academic background, coupled with his innate curiosity and vision, led him to become one of India’s foremost scientific minds. Throughout his illustrious career, Dr. Chidambaram played an instrumental role in shaping India’s nuclear capabilities, overseeing both the Pokhran-I (1974) and Pokhran-II (1998) nuclear tests, which cemented India’s position as a nuclear power on the world stage.
As a physicist, Dr. Chidambaram’s groundbreaking research in high-pressure physics, crystallography, and materials science greatly advanced the understanding of these fields. His pioneering work laid the foundation for modern materials science research in India, contributing to the nation’s scientific progress in multiple areas. His expertise in these complex disciplines not only bolstered India’s nuclear research but also advanced its technological prowess.
In addition to his work in nuclear weapons development, Dr. Chidambaram made significant strides in nuclear energy, ensuring that India remained at the forefront of scientific and technological advancements. As Director of the Bhabha Atomic Research Centre (BARC) and later as Chairman of the Atomic Energy Commission of India, he was integral to India’s peaceful nuclear energy initiatives. As Principal Scientific Adviser to the Government of India, Dr. Chidambaram guided national policies on defense, energy, and nuclear research, shaping the future of India’s scientific endeavors.
He was a vital member of the team that conducted India’s first nuclear test, Smiling Buddha, at Pokhran in 1974. His leadership during the Pokhran-II tests in 1998, which confirmed India’s nuclear deterrent, was a defining moment in the nation’s history. Chidambaram’s steadfast commitment to India’s defense and scientific advancement earned him respect both at home and abroad.
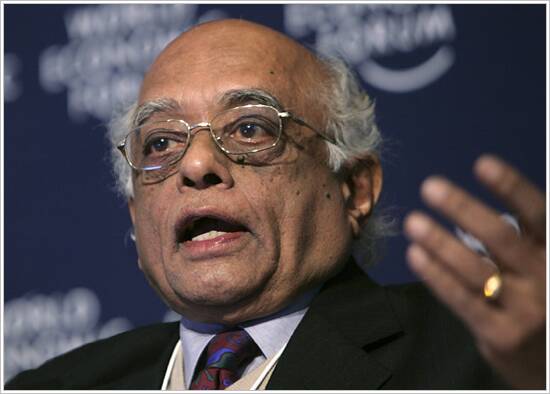
A visionary leader, Dr. Chidambaram believed in the power of science and technology to drive national development. His efforts were instrumental in championing key initiatives in energy, healthcare, and strategic self-reliance. He steered numerous projects that significantly advanced India’s science and technology landscape. Notably, he played a central role in the indigenous development of supercomputers and was the driving force behind the conceptualization of the National Knowledge Network, which connected research and educational institutions across India.
Dr. Chidambaram was also an ardent advocate for the application of science and technology to improve societal conditions. He established the Rural Technology Action Groups and the Society for Electronic Transactions and Security, among other programs. His emphasis on “Coherent Synergy” in India’s scientific efforts helped foster collaboration across various disciplines, accelerating the country’s scientific growth.
On the global stage, Dr. Chidambaram served as the Chairman of the Board of Governors of the International Atomic Energy Agency (IAEA) in 1994-1995 and contributed to several high-level international nuclear discussions. His expertise was sought worldwide, and in 2008, he was appointed to the Commission of Eminent Persons by the IAEA to assess the agency’s role in nuclear governance.
He was a vital member of the team that conducted India’s first nuclear test, Smiling Buddha, at Pokhran in 1974
In recognition of his exceptional contributions to science and national development, Dr. Chidambaram received several prestigious accolades, including the Padma Shri in 1975 and the Padma Vibhushan in 1999. He was also awarded honorary doctorates from several universities and was a fellow of several eminent Indian and international scientific academies.
Dr. Chidambaram’s passing marks the end of an era for India’s nuclear program and the global scientific community. His legacy as a scientist, visionary leader, and architect of India’s nuclear journey will continue to inspire future generations. His contributions to national security, energy, and technological innovation have left an indelible mark on India’s scientific and strategic landscape.
Rajagopala Chidambaram’s profound impact on India’s nuclear and scientific trajectory will be remembered for generations to come. His work in advancing both national defense and the peaceful use of nuclear energy stands as a testament to his vision of a self-reliant, scientifically empowered India.
“Deeply saddened by the demise of Dr Rajagopala Chidambaram. He was one of the key architects of India’s nuclear programme and made ground-breaking contributions in strengthening India’s scientific and strategic capabilities. He will be remembered with gratitude by the whole nation and his efforts will inspire generations to come,” Prime Minister Narendra Modi wrote on X.
Dr. Ajit Kumar Mohanty, Secretary, Department of Atomic Energy, in a statement issued, said, “Dr. Chidambaram was a doyen of science and technology whose contributions furthered India’s nuclear prowess and strategic self-reliance. His loss is an irreparable one for the scientific community and the nation.”
Space & Physics
The Story of the World’s Most Underrated Quantum Maestro
As the world celebrates the 131st birth anniversary of S.N. Bose, EdPublica explores the theoretical physicist’s unparalleled contributions to the field of quantum mechanics
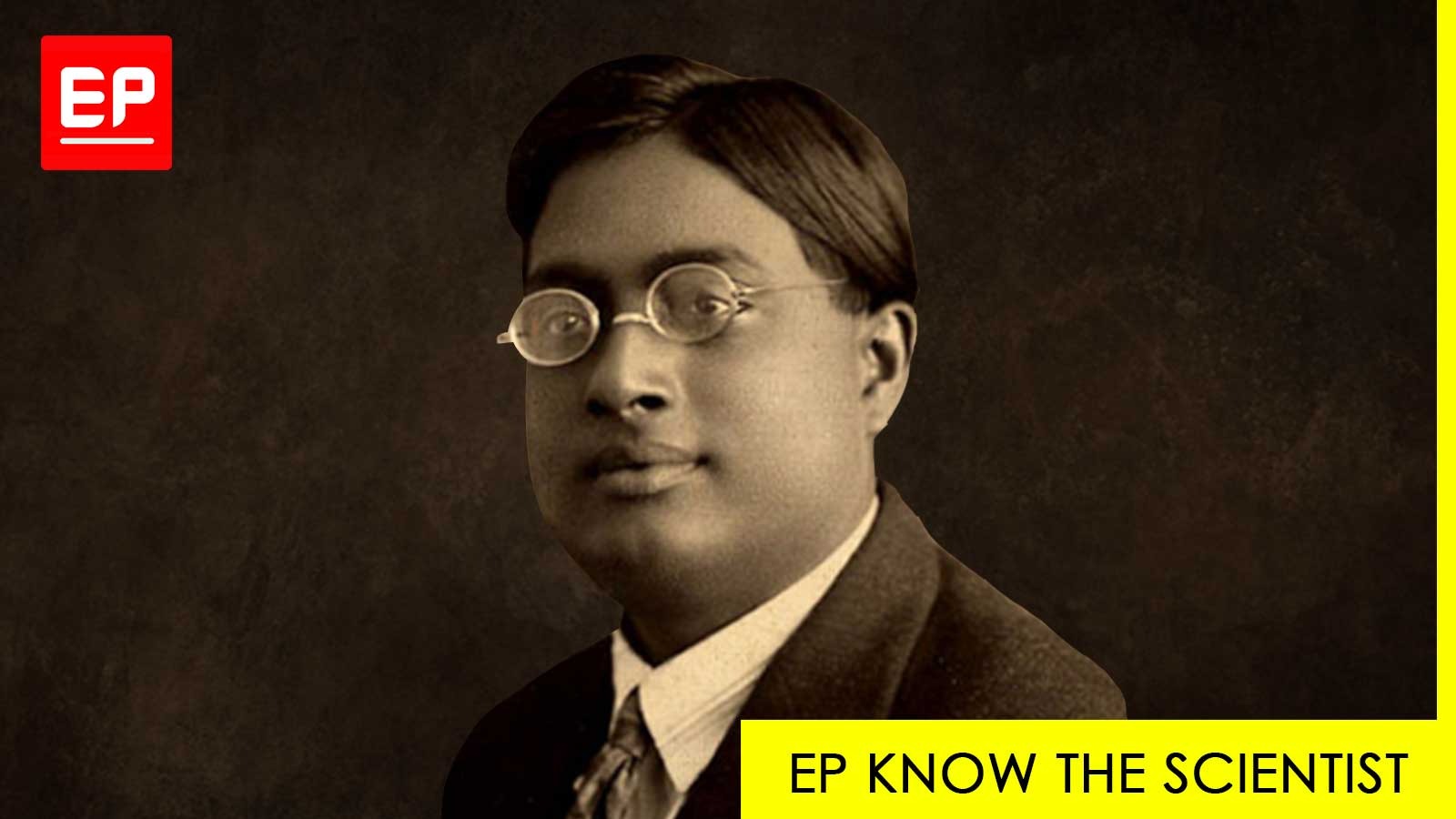
It’s 1924, and Satyendra Nath Bose, going by S.N. Bose was a young physicist teaching in Dhaka, then British India. Grappled by an epiphany, he was desperate to have his solution, fixing a logical inconsistency in Planck’s radiation law, get published. He had his eyes on the British Philosophical Magazine, since word could spread to the leading physicists of the time, most if not all in Europe. But the paper was rejected without any explanations offered.
But he wasn’t going to give up just yet. Unrelenting, he sent another sealed envelope with his draft and this time a cover letter again, to Europe. One can imagine months later, Bose breathing out a sigh of relief when he finally got a positive response – from none other than the great man of physics himself – Albert Einstein.
In some ways, Bose and Einstein were similar. Both had no PhDs when they wrote their treatises that brought them into limelight. And Einstein introduced E=mc2 derived from special relativity with little fanfare, so did Bose who didn’t secure a publisher with his groundbreaking work that invented quantum statistics. He produced a novel derivation of the Planck radiation law, from the first principles of quantum theory.
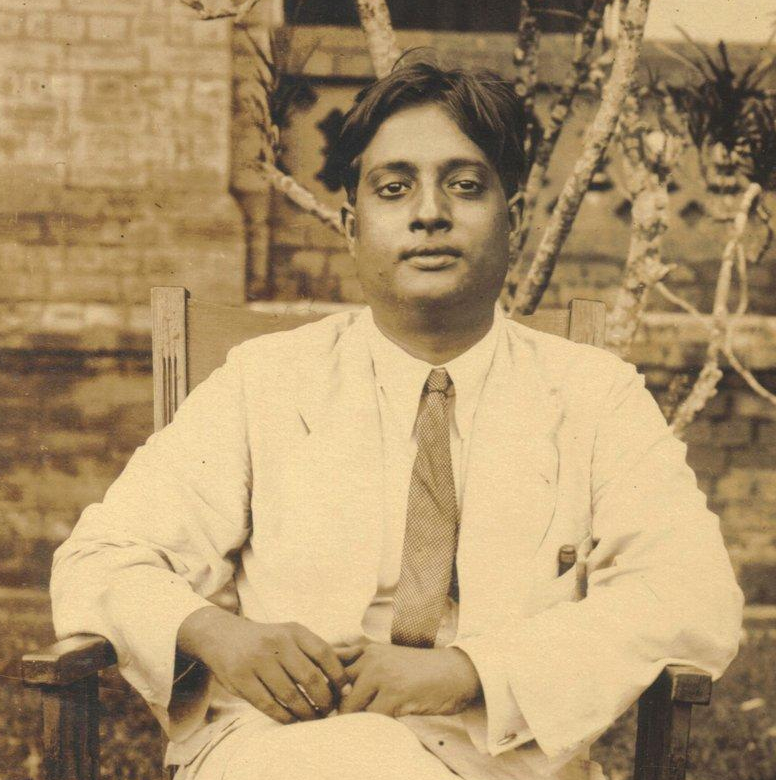
This was a well-known problem that had plagued physicists since Max Planck, the father of quantum physics himself. Einstein himself had struggled time and again, to only have never resolved the problem. But Bose did, and too nonchalantly with a simple derivation from first principles grounded in quantum theory. For those who know some quantum theory, I’m referring to Bose’s profound recognition that the Maxwell-Boltzmann distribution that holds true for ideal gasses, fails for quantum particles. A technical treatment of the problem would reveal that photons, that are particles of light with the same energy and polarization, are indistinguishable from each other, as a result of the Pauli exclusion principle and Heisenberg’s uncertainty principle.
Fascinatingly, last July marked the 100 years since Einstein submitted Bose’s paper, “Planck’s law and the quantum hypothesis” on his behalf to Zeitschrift fur Physik.
Fascinated and moved by what he read, Einstein was magnanimous enough to have Bose’s paper translated in German and published in the journal, Zeitschrift für Physik in Germany the same year. It would be the beginning of a brief, but productive professional collaboration between the two theoretical physicists, that would just open the doors to the quantum world much wider. Fascinatingly, last July marked the 100 years since Einstein submitted Bose’s paper, “Planck’s law and the quantum hypothesis” on his behalf to Zeitschrift fur Physik.
With the benefit of hindsight, Bose’s work was really nothing short of revolutionary for its time. However, a Nobel Committee member, the Swedish Oskar Klein – and theoretical physicist of repute – deemed it a mere advance in applied sciences, rather than a major conceptual advance. With hindsight again, it’s a known fact that Nobel Prizes are handed in for quantum jumps in technical advancements more than ever before. In fact, the 2001 Nobel Prize in Physics went to Carl Wieman, Eric Allin Cornell, and Wolfgang Ketterle for synthesizing the Bose-Einstein condensate, a prediction made actually by Einstein based on Bose’s new statistics. These condensates are created when atoms are cooled to near absolute zero temperature, thus attaining the quantum ground state. Atoms at this state possess some residual energy, or zero-point energy, marking a macroscopic phase transition much like a fourth state of matter in its own right.
Such were the changing times that Bose’s work received much attention gradually. To Bose himself, he was fine without a Nobel, saying, “I have got all the recognition I deserve”. A modest character and gentleman, he resonates a lot with the mental image of a scientist who’s a servant to the scientific discipline itself.
He was awarded the Padma Vibhushan, the highest civilian award by the Government of India in 1954. Institutes have been named in his honour, but despite this, his reputation has little if no mention at all in public discourse.
But what’s more upsetting is that, Bose is still a bit of a stranger in India, where he was born and lived. He studied physics at the Presidency College, Calcutta under the tutelage that saw other great Indian physicists, including Jagdish Chandra Bose and Meghnad Saha. He was awarded the Padma Vibhushan, the highest civilian award by the Government of India in 1954. Institutes have been named in his honour, but despite this, his reputation has little if no mention at all in public discourse.
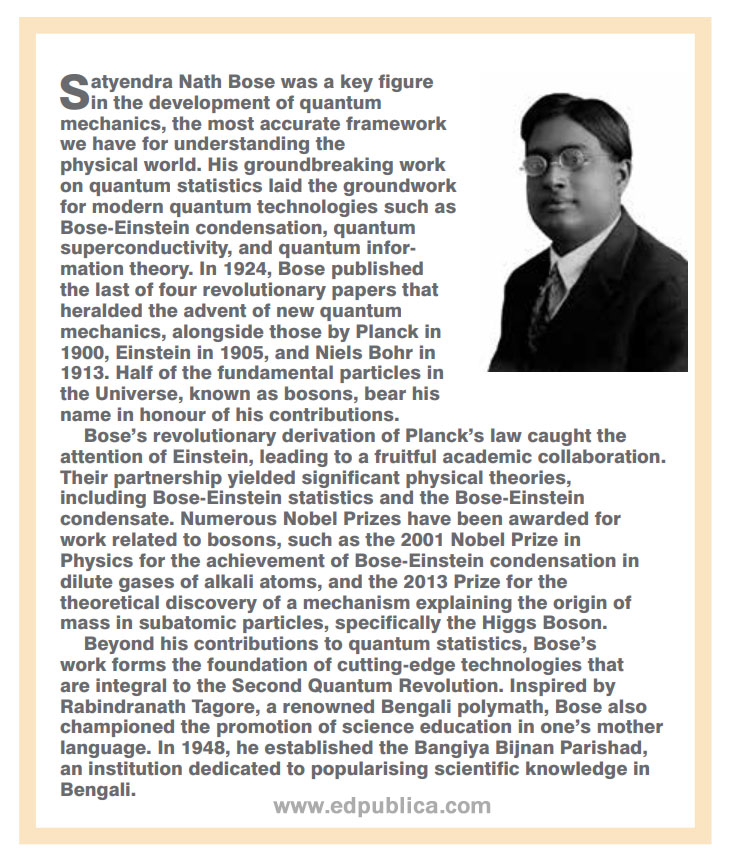
To his physicists’ peers in his generation and beyond, he was recognized in scientific lexicology. Paul Dirac, the British physicist coined the name ‘bosons’ in Bose’s honor (‘bose-on’). These refer to quantum particles including photons and others with integer quantum spins, a formulation that arose only because of Bose’s invention of quantum statistics. In fact, the media popular, ‘god particle’, the Higgs boson, carries a bit of Bose as much as it does of Peter Higgs who shared the 2013 Nobel Prize in Physics with Francois Euglert for producing the hypothesis.
Space & Physics
New Dust Models Shed Light on the End Stages of Sun-like Stars
New Insights into Hydrogen-Deficient Stars: Study of Planetary Nebula IC 2003 Reveals Key Evolutionary Clues
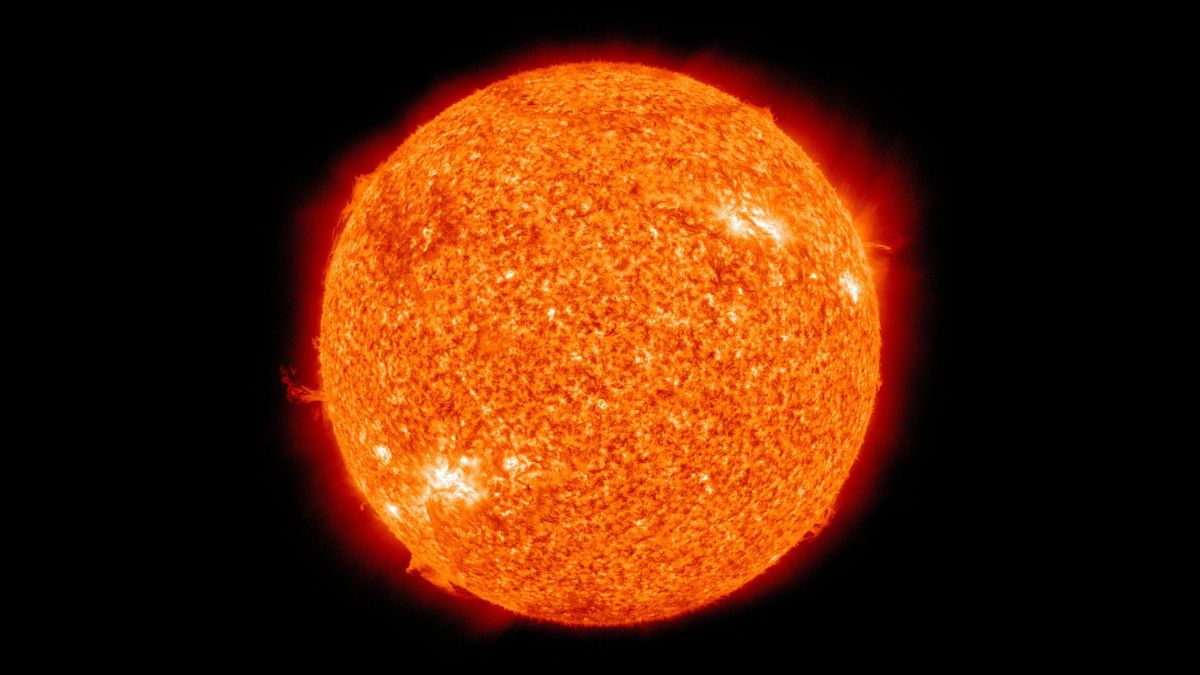
Careful modelling of the thermal and ionization structure of planetary nebulae, based on observations from the Vainu Bappu Telescope in Kavalur, Tamil Nadu, India, has enabled astronomers to deepen their understanding of the formation and evolution of these unusual hydrogen-deficient stars.
Planetary nebulae are shells of gas and dust ejected by stars like our Sun after they exhaust the hydrogen and helium fuel in their cores—a fate our Sun is expected to face in approximately 5 billion years. As the star’s core contracts due to the lack of nuclear fusion, it heats up and emits intense far-ultraviolet radiation. In the past, these stars appeared planet-like when viewed through small telescopes, a resemblance that led to their name.
While most stars in this late phase of their lives produce core remnants surrounded by tiny residual hydrogen envelopes, about 25% of them exhibit a deficiency of hydrogen and are instead rich in helium on their surfaces. Some of these stars also display strong mass loss and emission lines of ionized helium, carbon, and oxygen, characteristics identified as Wolf-Rayet (WR) features.
IC 2003, a rare planetary nebula, features a hydrogen-deficient central remnant star with WR characteristics.
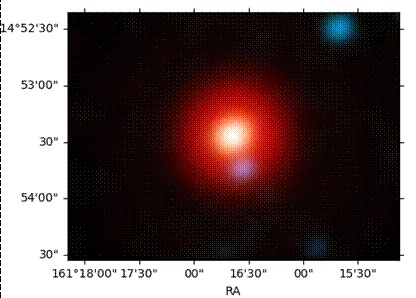
Although the evolutionary status of typical central stars of planetary nebulae is well-understood, the mechanisms behind the formation of hydrogen-poor stars remain largely unclear. The physical and chemical structures of the nebulae surrounding these stars provide valuable clues about their origin and evolution, making it essential to study their gas and dust in detail.
To investigate this further, astronomers from the Indian Institute of Astrophysics (IIA), an autonomous institute under the Department of Science and Technology, observed IC 2003 using the optical medium-resolution spectrograph (OMR) attached to the 2.3-meter Vainu Bappu Telescope at the Vainu Bappu Observatory in Kavalur, Tamil Nadu. “We also used ultraviolet spectra from the IUE satellite and broadband infrared fluxes from the IRAS satellite archives for this study,” said K. Khushbu, the lead author and Ph.D. student. These combined observations provided critical insights into the role of gas and dust in shaping the thermal structure of the nebula, ultimately enabling the team to derive precise parameters for the central star.
The models they used revealed that the nebula’s parameters, including the ionizing source’s mass and temperature, were significantly different from those predicted by dust-free models. “This study highlights the importance of dust grains in the thermal balance of ionized gas and helps explain the large temperature variations seen in nebulae, which are often key to resolving abundance discrepancies in astrophysical nebulae,” explained Prof. C. Muthumariappan, the supervisor and co-author of the study. “We used a one-dimensional dusty photo-ionization code, CLOUDY17.3, to simulate data from ultraviolet, optical, and infrared observations,” he added.
By modeling the photoelectric heating of the nebula caused by the dust grains, the researchers were able to replicate the thermal structure observed in the planetary nebula. “We even reproduced the large temperature gradient typically seen in nebulae with WR stars. Our determination of element abundances, such as helium, nitrogen, and oxygen, differs significantly from the values obtained empirically,” Khushbu noted.
The study also provided accurate grain size distributions within the nebula and highlighted the crucial role of photoelectric heating in explaining temperature variations. From their models, the researchers derived accurate values for the luminosity, temperature, and mass of the central star. They concluded that the star’s initial mass was 3.26 times that of the Sun, indicating it was a more massive star.
This research advances our understanding of the complex processes at work in the evolution of hydrogen-deficient stars and offers valuable insights into the origins of the unique features observed in planetary nebulae.
-
Society6 months ago
Death toll 280 & counting: what is the science behind Kerala’s deadly landslides?
-
Interviews6 months ago
‘Significant under-representation of black women in academic and research leadership’
-
Earth6 months ago
UFS and ARC establish research chairs to tackle climate change
-
Space & Physics4 months ago
Bubbles observed moving on a star for the first time
-
Society5 months ago
Rate of heart attacks and strokes was lower after COVID-19 vaccination: Study
-
Interviews6 months ago
‘Students with high emotional quotient better manage stress’
-
Learning & Teaching6 months ago
How AI is transforming India’s education System
-
Learning & Teaching5 months ago
India’s Premier Universities Ranked: Indian Institute of Science tops the list