Space & Physics
Starship reaches farther than ever in latest ‘test’
The latest launch saw the Starship rocket travel the farthest it has ever gone, streaking for 50 minutes through the vast layers of the atmosphere before reaching space.
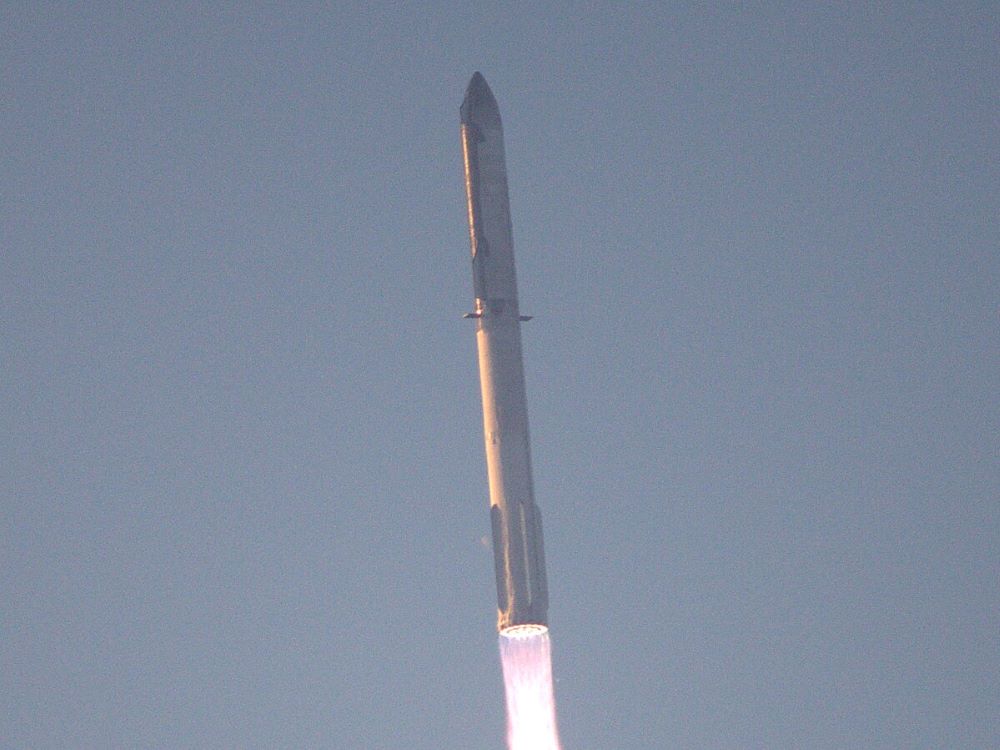
Earlier today, SpaceX’s Starship super-heavy lift rocket successfully arrived in outer space. However, it burnt upon atmospheric re-entry during the return of its reusable rocket stages.
Starship’s vital in Elon Musk’s long-stated vision to get humans to Mars.
The latest launch saw Starship travel the farthest it has ever gone, streaking for 50 minutes through the vast layers of the atmosphere before reaching space.
The latest launch saw the Starship rocket travel the farthest it has ever gone, streaking for 50 minutes through the vast layers of the atmosphere before reaching space.
Musk, on X, confirmed the rocket reached ‘orbital velocity’ meaning it’s engines achieved the necessary speed to arrive in orbit.
The launch was part of a series of ‘tests’, according to SpaceX’s own communique, meaning these rocket launches are to help supplement data to improve Starship’s features.
The previous missions lasted 4 and 8 minutes respectively, without reaching space.
Given Starship’s upper stage and booster are reusable, it can be made to land back at the spaceport, or even a drone ship at sea.
In this test however, SpaceX planned a ‘splashdown’ instead. However, the rocket stages burnt upon atmospheric re-entry, ending the test early.
SpaceX and Elon Musk, its founder and CEO were positive about the test.
The launch was part of a series of ‘tests’, according to SpaceX’s own communique, meaning these rocket launches are to help supplement data to improve Starship’s features.
Meanwhile, Starship has gotten ever closer to being the heaviest and most powerful reliable rocket ever built.
Following the launch, Musk posted twice on X, ecstatically stating that “Starship will take humanity to Mars,” and make life “multiplanetary.”
Space & Physics
Moon’s clocks are ticking faster than Earth’s
A new study reveals that Moon’s clocks are ticking faster than Earth’s, and it offers implications for new angles to the space exploration
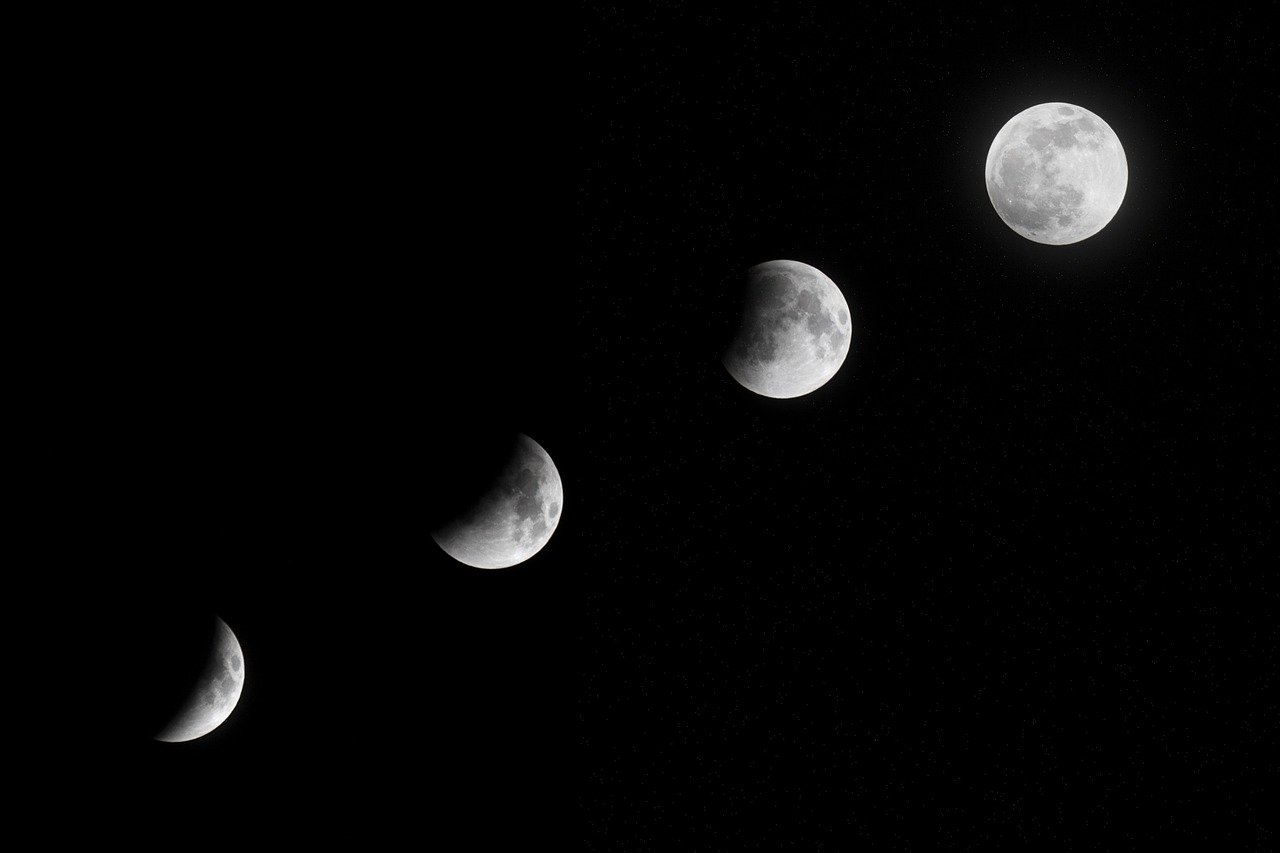
Clocks on the Moon tick slightly faster than those on Earth, a new study reveals. It has significant implications for future space missions. Specifically, clocks placed near the Moon gain an additional 56.02 microseconds per day compared to those on Earth. Thanks to Albert Einstein. This phenomenon can be attributed to Einstein’s theory of relativity, which states that both gravity and speed influence the passage of time.
Einstein’s theory states that time is not a constant; it varies depending on the strength of gravitational fields and the speed at which an object is moving. The study highlights how this effect plays out in the Moon’s environment, where the weaker gravitational field compared to Earth causes time to pass slightly faster.
As humanity prepares for more frequent missions to the Moon, Mars, and other celestial bodies, understanding these variations in timekeeping becomes crucial. The time discrepancies, although minute, can impact everything from navigation and communication to the synchronization of systems across multiple platforms. Space missions rely on precise timing to maintain their operations, and any slight difference in time could have significant consequences.
The Lagrange Points
The study, published in The Astronomical Journal, also points out the behaviour of clocks positioned at Lagrange points—specific regions in space where gravitational forces from two large bodies, like Earth and the Moon, balance out. These points are particularly important for future space exploration as they could serve as staging areas or refueling stations for spacecraft traveling between Earth and the Moon.
Lagrange points offer stable environments for spacecraft to dock, making them prime candidates for mission planning. As such, understanding time at these locations is essential to ensure synchronization between spacecraft and lunar bases.
Time Matters
Time is a critical factor in space exploration for several reasons. First, it underpins the functioning of communication systems. Long-distance communication between Earth and distant missions often faces delays due to the vast distances involved. Additionally, varying gravitational effects between locations in space can lead to further time discrepancies. Understanding how time behaves in these contexts ensures that systems can be adjusted accordingly to maintain reliable communication.
Second, precise timing is essential for navigation. Spacecraft must be carefully synchronized to avoid collisions and to successfully carry out maneuvers, such as landing on the Moon or Mars. Without accurate timekeeping, the success of these operations could be jeopardized.
Finally, as lunar bases and spacecraft rely on different timing systems, harmonizing these clocks will be essential for smooth operations. Coordination between various space systems, both human and robotic, will be vital as we push further into space exploration.
Know The Scientist
Pierre Curie: The precision of a scientific pioneer
Pierre Curie is perhaps best known for his work on magnetism
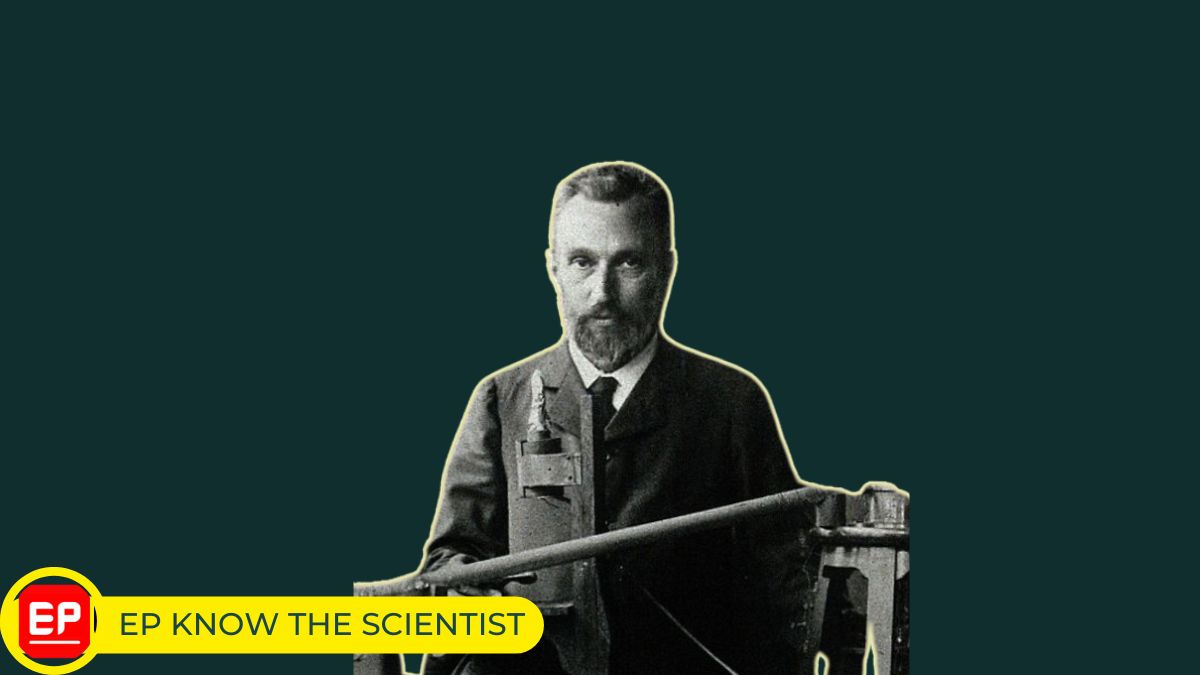
Pierre Curie (1859–1906) was a man whose legacy has shaped the course of modern science, yet his name is often overshadowed by that of his famous wife, Marie Curie. Despite this, Pierre’s contributions to physics, particularly in the field of magnetism and the discovery of radioactivity, were revolutionary and continue to influence scientific research today.
Early Life and Education
Born in Paris on May 15, 1859, Pierre Curie grew up in an intellectually stimulating environment. His father, Eugene, was a physician, and his mother, Sophie, was a teacher, which cultivated in Pierre a deep passion for learning. From an early age, Pierre showed an exceptional aptitude for mathematics and physics, subjects that would later define his career.
By the time Pierre was 16, he had already completed his studies in mathematics and physics, earning a degree from the prestigious Sorbonne University in Paris. This early foundation in scientific inquiry laid the groundwork for his future innovations.
In 1895 together with his brother Jacques Curie, Pierre Curie developed the Curie point—the temperature at which certain magnetic materials lose their magnetism
Innovative Work in Magnetism and Crystallography
Pierre Curie is perhaps best known for his work on magnetism. In 1895, together with his brother Jacques Curie, he developed the Curie point—the temperature at which certain magnetic materials lose their magnetism. This work, foundational in the study of thermodynamics and magnetism, continues to be a key concept in modern physics.
Additionally, Pierre Curie’s research in crystallography and his study of the magnetic properties of materials played a pivotal role in the development of solid-state physics. His work laid the foundation for understanding the relationship between a material’s structure and its magnetic properties, which remains essential in materials science today.
The Discovery of Radioactivity
However, Pierre Curie’s most significant contribution came from his work on radioactivity, which would forever alter the understanding of matter itself. In the late 19th century, the mysterious rays emitted by certain substances, like uranium, intrigued scientists. Working alongside his wife, Marie Curie, Pierre embarked on a series of experiments to better understand this phenomenon.
Their work, starting in 1898, led to the discovery of two new elements: polonium and radium. Marie Curie coined the term “radioactivity” to describe the spontaneous emission of radiation from these elements, but it was Pierre’s precise experimental methods and scientific rigor that helped bring clarity to the phenomenon. Their discovery of radium, in particular, was a breakthrough that would lead to numerous advancements in medical treatments, including cancer therapy.
Nobel Recognition and Collaboration with Marie Curie
In 1903, Pierre Curie, together with Marie Curie and Henri Becquerel, was awarded the Nobel Prize in Physics for their joint work on radioactivity. The recognition marked the first time a Nobel Prize had been awarded to a couple. However, what makes this achievement particularly notable is that Pierre Curie insisted that Marie be included in the award, a gesture that demonstrated not only his scientific partnership with his wife but also his support for women in science, a rare stance in the male-dominated field of the time.
Tragically, Pierre Curie’s life was cut short in 1906 when he was killed in a street accident at the age of 46
Pierre Curie’s dedication to scientific rigor and his ability to work collaboratively with Marie, his wife and fellow scientist, was vital to their success. Their work would not only earn them the Nobel Prize but also set the stage for later advancements in nuclear physics and medicine.
Tragic Loss and Enduring Legacy
Tragically, Pierre Curie’s life was cut short in 1906 when he was killed in a street accident at the age of 46. His death was a blow to both the scientific community and his family. However, his legacy continued through his wife, Marie, who carried on their groundbreaking work and became the first woman to win a second Nobel Prize.
Today, Pierre Curie is remembered as a visionary physicist whose discoveries were instrumental in shaping the fields of physics, chemistry, and medicine. His contributions to magnetism, crystallography, and radioactivity remain foundational to scientific inquiry. His work continues to inspire scientists across disciplines and serves as a reminder of the power of precision, collaboration, and dedication in the pursuit of knowledge.
Space & Physics
MIT Physicists uncover key Mechanism behind fractional charge in Graphene
In the decades-long history of studying these phenomena, no one has observed a system that naturally leads to such fractional electron effects, according to the researchers.
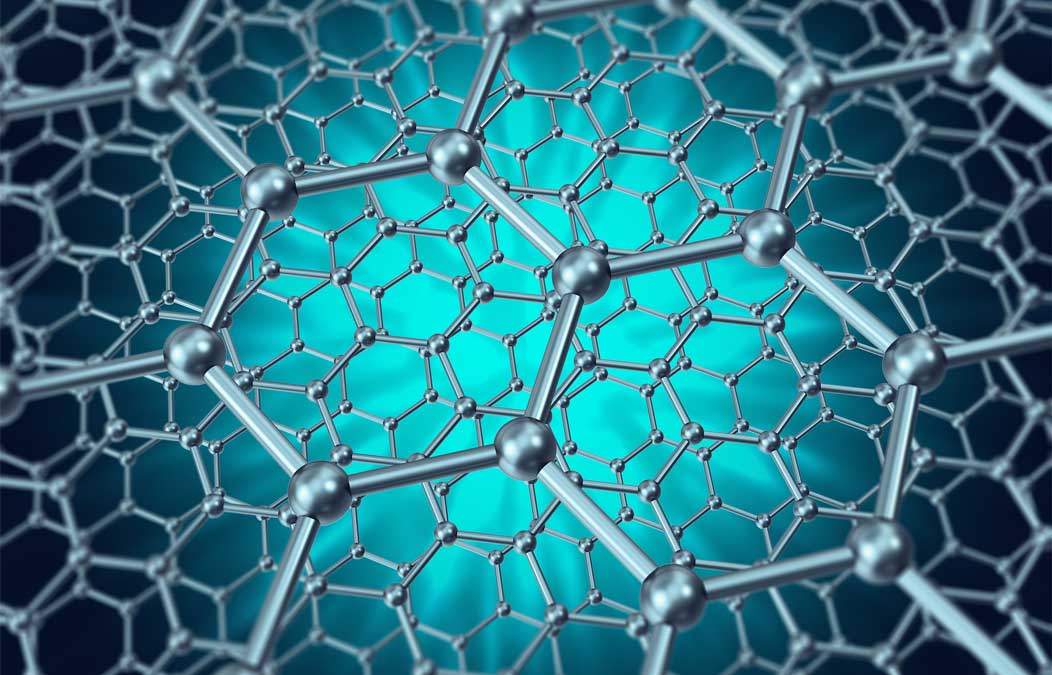
MIT physicists have made a significant breakthrough in understanding the phenomenon where electrons split into fractions of their usual charge, offering new insights into the behaviour of exotic electronic states in graphene and other two-dimensional materials.
This latest research builds on a discovery earlier this year, when a team led by Assistant Professor Long Ju at MIT reported that electrons in pentalayer graphene—a structure composed of five graphene layers stacked on top of boron nitride—exhibited fractional charge. Remarkably, this behaviour was observed without the application of a magnetic field, challenging prior assumptions.
Previously, scientists knew that under a strong magnetic field, electrons could split into fractions as part of the fractional quantum Hall effect. However, Ju’s findings marked the first time such fractional behaviour occurred in graphene without any magnetic influence, which led to the coining of the “fractional quantum anomalous Hall effect.” Since then, researchers have been eager to uncover how fractional charge could emerge in this unusual system.
MIT professor Senthil Todadri, who led the new study published in Physical Review Letters, offers a critical piece of the puzzle. Through detailed quantum mechanical calculations, Todadri and his team discovered that the electrons in pentalayer graphene form a crystal-like structure, which provides the ideal conditions for fractional electron behavior.
“This is a completely new mechanism,” said Todadri. “In the decades-long history of studying these phenomena, no one has observed a system that naturally leads to such fractional electron effects. It opens the door to all kinds of new experimental possibilities.”
The study, which includes contributions from Zhihuan Dong and former postdoc Adarsh Patri, is part of a wider body of research. Two other teams—one from Johns Hopkins University and another from Harvard University, UC Berkeley, and Lawrence Berkeley National Laboratory—have also reported similar findings in the same journal issue.
Building on “Twistronics” and the Magic-Angle Graphene Discovery
This research builds upon the work of MIT physicist Pablo Jarillo-Herrero and his team, who in 2018 were the first to demonstrate that twisting two sheets of graphene could give rise to novel electronic behaviors. This discovery of “magic-angle graphene” spurred a new field known as “twistronics,” focused on understanding how the interactions between twisted two-dimensional materials could lead to unusual quantum phenomena, such as superconductivity and insulating behavior.
“We quickly realized that these twisted systems could provide the right conditions for fractional electron phenomena to emerge,” said Todadri, who collaborated with Jarillo-Herrero on a 2018 study that theorized such systems might exhibit fractional charge without a magnetic field. “We saw these systems as ideal platforms to study these fractional effects.”
A Surprising Discovery and the New Crystal Model
In September 2023, Todadri received an unexpected call from Ju, who was eager to share data showing fractional charge behavior in pentalayer graphene. This discovery caught Todadri by surprise, as it did not align with his earlier predictions. In his 2018 paper, Todadri had theorized that fractional charge would emerge from a specific twisting of the electron wavefunction, and that this twisting would intensify as more graphene layers were added.
“Initially, we expected the wavefunction to wind five times in pentalayer graphene,” Todadri explained. “But Ju’s experiments showed that it only wound once. This raised a big question—how do we explain what we’re seeing?”
Uncovering the Electron “Crystal”
Todadri and his team revisited their hypothesis and discovered they had overlooked an important factor. The conventional approach in the field had been to treat electrons as independent entities and analyze their quantum properties. However, in the confined, two-dimensional space of pentalayer graphene, electrons are forced to interact with each other, behaving according to their quantum correlations in addition to their natural repulsion.
By incorporating these interelectron interactions into their model, the team was able to match their predictions with the experimental data Ju had obtained. This led them to a crucial realization: the moiré pattern formed by the stacked graphene layers induces a weak electrical potential that forces the electrons to interact and form a crystal-like structure. This electron “crystal” creates a complex pattern of quantum correlations, allowing for the formation of fractional charge.
“The crystal has a whole set of unique properties that differentiate it from ordinary crystals,” said Todadri. “This opens up many exciting avenues for future research. In the short term, our work provides a theoretical foundation for understanding the fractional electron observations in pentalayer graphene and predicting similar phenomena in other systems.”
This new insight paves the way for further exploration into how graphene and other two-dimensional materials might be used to engineer new electronic states, with potential applications in quantum computing and other advanced technologies.
-
Society4 months ago
Death toll 280 & counting: what is the science behind Kerala’s deadly landslides?
-
Space & Physics6 months ago
Fusion Energy: The quest for unlimited power
-
Interviews4 months ago
‘Significant under-representation of black women in academic and research leadership’
-
Learning & Teaching5 months ago
Delving into the historical perspective of learning
-
Earth5 months ago
Ancient Earthquake Redefined Ganges’ Flow Through History
-
Earth5 months ago
The place where seabirds outnumber people
-
Society5 months ago
Reborn; India’s 1600-year-old Ivy League University
-
Earth5 months ago
Rate of global warming caused by humans at an all-time high